Innovative Synapse Ablators Developed to Enhance Understanding of Neural Circuits
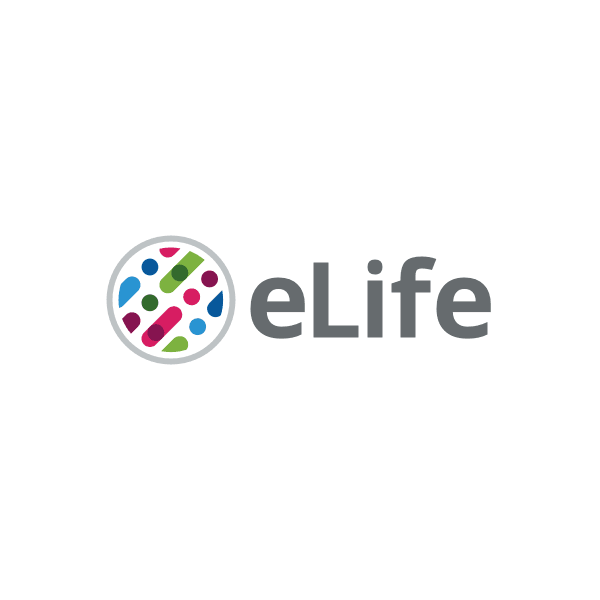
In a groundbreaking study, researchers have introduced three novel synapse ablation tools designed to enhance the understanding and manipulation of neural circuits. These tools include PFE3, which specifically ablates excitatory synapses; paGFE3, a photoactivatable variant of the inhibitory synapse ablator; and chGFE3, a chemically activated version of GFE3. The development of these tools allows for precise targeting of specific postsynaptic sites, enabling researchers to disrupt neural circuits with remarkable specificity.
PFE3 is constructed with three distinct functional domains. The E3 ligase Mdm2 facilitates the ubiquitination of PSD-95, a critical protein associated with excitatory synapses. The PIR domain subsequently recruits proteasomes to the synapse to degrade the ubiquitinated PSD-95 protein. Both of these domains are directed towards PSD-95 via a specialized binding protein known as PSD-95.FingR. This innovative design is rooted in previous research that demonstrated the coordinated action of Mdm2 and PIR from PCDH 10 plays a significant role in the degradation of excitatory synapses (Tsai et al., 2012).
The efficacy of PFE3 was substantiated by experiments that showed efficient degradation of PSD-95 and subsequent ablation of synapses could only occur when all three components of PFE3 were present. The expression of PFE3 resulted in a highly effective ablation of PSD-95 in cultured cortical neurons, effectively blocking excitatory synaptic transmission in retinal ganglion cells when tested in vivo.
This efficient elimination of excitatory synapses was observed within 48 hours of PFE3 expression, contrasting sharply with findings from studies involving PSD-95 knockout mice. In those models, reductions in AMPA receptors and overall excitatory transmission were either negligible or minimal, depending significantly on the developmental stage of the mice. One potential explanation for this discrepancy may be the upregulation of SAP-102, a MAGUK protein that shares high homology with PSD-95, in PSD-95 knockout models. Such compensation could mask the effects of PSD-95 absence (Coley and Gao, 2019).
Interestingly, PSD-95.FingR also labels SAP-1023, suggesting that PFE3 could effectively degrade SAP-102, which might otherwise compensate for the loss of PSD-95. This indicates that the binding mechanism of PSD-95.FingR to MAGUK homologs, such as SAP-102 and SAP97, could facilitate a more efficient and widespread ablation of excitatory synapses compared to if it were limited to PSD-95 alone. Additionally, it raises questions about the speed of compensatory transcriptional changes. Such changes could be slow to respond or might react specifically to alterations in mRNA levels rather than protein levels.
Future research utilizing PFE3 may help to further explore the mechanisms of compensation that maintain synaptic homeostasis during fluctuations in protein levels. One particularly intriguing application of PFE3 is investigating the role of PSD-95 in preserving synaptic structure. Questions arise about its relationship with dendritic spines and the dynamic changes that occur in synapses before and after the ablation of PSD-95. While PSD-95 puncta are known to regenerate in cultured neurons, the behavior of these structures in mature neurons in vivo remains poorly understood. Would they reconnect with their original presynaptic partners, and how would this affect neural circuit functions post-regrowth? Another area of interest is whether prolonged PFE3 expression could lead to permanent alterations in circuit connectivity.
Moreover, the researchers have enriched GFE3 with temporal and spatial control features, enabling its activation through light (paGFE3) or chemical means (chGFE3). This was achieved through a standard method that fuses two distinct domains essential for protein function with separate components of a light- or chemically-triggered protein complex. Although this method has previously shown success in regulating various biological processesincluding transcription, neurotransmitter release, and gene editingit faced challenges with background activation in photoreceptive complexes that rendered them unsuitable for the highly potent GFE3.
As a solution, the team developed a novel photoactivatable complex called PhLIC, which is based on the photocleavable protein PhoCl and a 10-mer known as PBP. Remarkably, PhLIC exhibited no measurable background activation during tests with paGFE3. This characteristic makes paGFE3 particularly well-suited for use in transgenic models, including mice and zebrafish, where prolonged inertness before activation is crucial. By expressing paGFE3 in specific driver lines such as Gal4 (in zebrafish) or Cre (in mice), researchers can achieve precise ablation of inhibitory synapses in designated cell types with exceptional temporal control, all without the need for viral injections. Moreover, the controlled application of 400 nm light could enable the ablation of inhibitory synapses with single-cell spatial resolution.
Furthermore, the PhLIC complex presents exciting prospects for developing photoactivatable variants of other proteins beyond GFE3. For example, it could be utilized to engineer split versions of potent toxins, allowing for targeted cellular alterations at specific times without any background activity. On the other hand, chGFE3 operates on a system akin to those used for degrading exogenous proteins with eDHFR tags (Etersque et al., 2023). While similar in efficiency and reversibility to paGFE3, chGFE3 specifically targets endogenous Gephyrin to ablate inhibitory synapses.
Notably, both paGFE3 and chGFE3 share the advantage of exhibiting no background activity. While chGFE3s utility overlaps with that of paGFE3, it is particularly valuable in scenarios where photoactivation may be cumbersome or impractical. For instance, if target cells are distributed over a larger area or when a sustained ablation is required, chGFE3 becomes more advantageous. However, one potential drawback of using chGFE3 in vivo is the requirement for injecting the chemical dimerizer TH, which may necessitate systemic administration, especially since both components of TH have been shown to penetrate the brain effectively when injected systemically (Mohar et al., 2022; Ineichen et al., 2020).
In conclusion, the development of these innovative proteins for the ablation of excitatory and inhibitory synapses marks a significant advancement in neuroscience. These tools not only offer the potential to dissect the intricate structure and function of neural circuits but also pave the way for future explorations into the underlying mechanisms of synaptic compensation and connectivity.